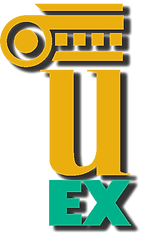
CATALYST PREPARATION AND THEIR APPLICATION TO REMOVE REFRACTORY WASTEWATER CONTAMINANTS BY MEANS OF CATALYTIC AND PHOTOCATALYTIC OXIDATION PROCESSES
CTQ2012/35789/c02
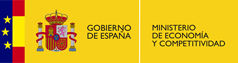
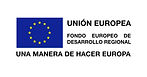
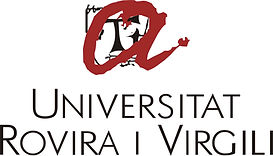
UEX SUBPROJECT BACKGROUND
PHOTOCATALYTIC OZONATION
SUBPROJECT CTQ2012/35789/C02/01
Results from studies of hydrogen peroxide formation from the breakdown of water molecules was the origin of photocatalytic oxidation, the simultaneous use of some photocatalyst (semiconductor) and radiation, and its further use in water pollutant removal. Fujishima and Honda (1972) were the first who proposed this process but for energetic purposes with an electro-photochemical cell, inert cathode and titania anode (rutile). Years later, Frank and Bard (1977) first applied the photocatalytic oxidation by using TiO2 and solar light, to remove cyanide and sulfite from water. From this point, application of photocatalytic oxidation to remove water pollutants has attracted the attention of numerous researchers as shown in many published works on contaminants removal from air and water in scientific journals, books and reviews (Gaya y Abdullah, 2008, Augugliaro et al., 2006, Oppenländer, 2003; De Lasa et al., 2005). Thus, Turchi and Ollis (1990) proposed the use of this process: radiation, one semiconductor (TiO2) and one oxidant (oxygen or air) to generate hydroxyl free radicals, that is, as AOP. Photocatalytic oxidation is based on the excitation of a semiconductor by a light radiation with energy greater than the necessary to allow the jumping of electrons of the valence band of the semiconductor to the conduction band (band gap). So strong oxidant holes are created (h+) while the conduction band becomes negatively charged (e-). The obtained positive sites with enough oxidant capacity (redox potential more positive than the corresponding to the H2O/OH. system), can transform the adsorbed water molecules or hydroxyl ions into hydroxyl radicals or to directly act on the dissolved organic matter, both processes being practically undistinguishable. Nonetheless, the process presents as main drawback the recombination reaction between electrons and positive centers, which inhibits the rate process because of a low concentration of hydroxyl radicals. For solving this problem the oxidation is carried out in the presence of an oxidizing agent capable of trapping electrons and prevent their return to the valence band and the recombination. To do this, the oxidant must have a more positive redox potential than that of the semiconductor conduction band. Thus, the superoxide ion radical, O2.-, is formed and protonated to yield the hydroperoxide ion radical, HO2., with further formation of hydrogen peroxide. This oxidant can also acts as electron-trapping agent. Because of its low cost, stability, non-toxicity, insolubility and the redox potential of their valence and conduction bands, titanium dioxide is the semiconductor catalyst more widely used. The most common application is in powder form (nanometer size) since in this way a higher surface is exposed to radiation and then pollutant removal is also higher. However, the nanometer size is one of the problems for real application since separation from water is difficult and expensive requiring different methods: pH adjustment, coagulation-flocculation-sedimentation and frequently further microfiltration (Watts and Kong, 1995). A second drawback is the need of using radiations of wavelength lower than 387 nm since the band gap is 3.2 eV. Although according to these data, UVA radiation is enough to promote charge separation in TiO2, the use of radiations of lower energy (visible spectrum of light) is more recommended from environmental sustainability reasons. One of the advantages of TiO2, however, is that oxygen can be used for trapping electrons since the redox potential of the O2/O2.- system is more positive than the one of the TiO2 conduction band. Both negative points, difficult separation from water and the possibility of using radiation of lower energy closer to the visible zone of spectrum or the own visible zone are the reasons of the subjects in recently works on photocatalytic oxidation. Regarding the use of radiations of lower energy, the literature shows numerous works on the preparation of catalysts with band gap lower than 3.2 eV. Many of these catalysts are based on TiO2. Thus, different forms of catalysts have been investigated such as cation (Fe, Cu, Rh, Mo, V, etc.) or anion (N, C, S, F) doping. On the whole, the presence of a doping cation, which is incorporated into the crystalline structure of TiO2, has shown an increase of visible radiation absorption because of the formation of an intermediate energy level in the TiO2 band gap (Litter, 1999). Visible radiation can excite the electron of intermediate energy level to the conduction band. In addition, recombination could be partially avoided since the doping metal could act as a trap of electrons and positive centres (Malato et al., 2009, Litter, 1999).On the other hand, doping anions have also shown to increase the visible light absorption due to a decrease of the band gap of the semiconductor, appearance of an intermediate level of energy and/or the presence of oxygen vacancies, (Malato et al., 2009, Chatterjee and Dasgupta, 2005). In this sense, application of this type of catalysts in photocatalytic ozonation would be a feasible way that, in any case, would favor the absorption of visible light.Another strategy to improve the absorption of visible light is the synthesis of composite materials of two semiconductors, generally, by coupling TiO2 and a second semiconductor with lower band gap (Malato et al., 2009). These materials are oxide mixtures such as TiO2-WO3. The conduction band of WO3 is less negative tan that of TiO2. So, the electrons can be transferred from the conduction band of TiO2 to the conduction band of WO3, yielding a more efficient charge separation (e-/h+) (recombination is avoided or reduced) (Keller and Garin, 2003; Dan et al., 2011). Other studies on catalyst preparation to form oxide mixture of Ti and In, Fe, Al, Zr, Sn, etc have been carried out (Shchukin et al., 2004). In these works, the main variables investigated were the percentage of oxide introduced on the TiO2, the photocatalytic activity of the new material as well as the optical absorption, the specific surface, acidity, oxygen reduction, etc.In addition to TiO2, the preparation and application of other semiconductors of lower band gap have also been investigated. Here, catalysts such as WO3 (2,8 eV), commented above, that theoretically can be excited with radiation of 443 nm, corresponding to the visible zone of the electromagnetic spectrum, (Abe et al., 2008), as well as other inorganic compounds like ZnO, ZnS, CdS (Hernández-Alonso y col., 2009) have been studied. However, the conduction band of WO3 has a redox potential more positive than oxygen. So, the charge recombination is an important problem. The CdS, in particular, has a band gap of 2.4 eV. Consequently, the radiations up to 517 nm could produce charge separation and initiate photocatalytic oxidation. However, the problems with the stability of the active phase due to metal leaching have been observed and these latter catalysts do not seem to be a feasible solution in spite of its capacity for the absorption of visible light (Hernández-Alonso y col., 2009).Regarding the separation of the catalyst from water after the oxidation process, literature also reports works on the preparation and application of TiO2 supported in different materials such as glass or aluminum sheets, ceramic tiles, etc. (Chen et al., 2006, Kemmitt et al., 2004, Macedo et al., 2007). Since the coating is very thin the active surface of the catalyst is very low compared to the bulk catalyst and the efficiency of the process is reduced up to 60-70% when compared with titania in powder form (Kabra et al., 2004). However, some different results have been obtained (Kim et al., 2005). Another more novel possibility is the use of a catalyst with magnetic properties. In this case, an external magnetic field can separate the catalyst from the water after the reaction process (Belessi et al., 2010, Álvarez et al., 2010).Practically, in almost all the works quoted here, oxygen was the oxidizing agent used. More recently, studies on photocatalytic ozonation have been developed, that is, the same process of photocatalytic oxidation but simultaneously using ozone-oxygen instead of oxygen. In the last 6 years (since 2005) the data base of Scopus shows 42 publications on photocatalytic ozonation (12 of them from the UEX research group) and 1426 papers when the key words used are photocatalytic oxidation. This difference clearly shows the development of both processes. Today, photocatalytic oxidation studies mainly focus on the synthesis of catalysts that allow the use of radiations of lower energy corresponding to the visible region of electromagnetic spectrum of light and their separation from water, while photocatalytic ozonation is in an incipient phase of research although with potential possibilities. Thus, from the basic reactions of the mechanisms of ozonation and photocatalytic oxidation it seems evident that ozone, of stronger oxidizing character than oxygen, can more efficiently trap electrons in the conduction band of semiconductors and, in addition, generate a high concentration of hydroxyl radicals. Another possible and positive effect is that ozone can favor the use of semiconductors such as WO3 since the conduction band is more negative than the O3/O3.- system and, as a consequence, charge recombination can be reduced and more hydroxyl radicals can be formed.In the photocatalytic ozonation studies so far carried out, basically with TiO2 (either in powder or in fibers or in some cases forming a composite material with carbon black) a significant increase of the oxidation rate of different contaminants is observed compared to that from the application of photocatalytic oxidation (Agustina y col., 2005, Augugliaro y col., 2006). However, compared to single ozonation, kinetic differences are only slightly more favorable for the photocatalytic ozonation process, than for ozone-free process. These low kinetic differences observed in some cases between single ozonation and photocatalytic ozonation are due to the nature of treated contaminants. Furthermore, as the UEX group has demonstrated in a previous project (Project CTQ2009/13459/C05/05), for fast ozone reacting contaminants, simultaneous application of photocatalytic oxidation does not seem a recommended option from a practical point of view. This effect was observed using different pharmaceutical compounds, phenols, amines, etc. However, when these compounds are eliminated, the formed intermediates are usually refractory for the direct reaction with ozone. In this case, the photocatalytic ozonation is a good process for the removal of these high refractory organic compounds. In this sense, this technology can also be applied to the treatment of urban and industrial wastewater that contains refractory compounds. In previous studies (see section 6 below), photocatalytic ozonation using titania as catalyst showed to be the best technology, among the processes studied, to obtain the highest reductions of COD or TOC. Nonetheless, there are numerous contaminants, in addition to wastewater, classified as priority (with maximum contaminant level established by environmental organisms) or emergent, that is, pharmaceuticals, products for personal care, etc, and potentially toxic, that are refractory to the ozone attack. Among these refractory contaminants, there are pesticides such as 2,4-D, t-butylazine, N,N-Diethyl-meta-toluamide, etc, aromatic hydrocarbons such as nitrobenzene, BTX group, fluorene, etc, phthalic acid derivatives, and also pharmaceuticals or used in medicine such as clofibric acid, iopromide, diazepam, etc. Removal of these compounds from water is of great environmental interest, especially if they are present in wastewaters to be reused. Therefore, AOPs should be applied to remove this kind of contaminants since some tertiary treatment processes such as single ozonation is enough to eliminate non refractory compounds. Finally, it should be highlighted that an important number of works so far conducted with ozone alone or combined with catalyst and/or radiation have focused on contaminants that really react in a fast way with ozone such as some pharmaceuticals, dyes, phenols, humic acids, etc, while in the few cases where refractory contaminants (i.e. some pesticides) have been treated with photocatalytic ozonation. In these works TiO2, in powder form or in fibers, was mainly the only photocatalyst used.